A study of the shortwave schemes in the Weather Research and Forecasting model
Testo completo
Figura
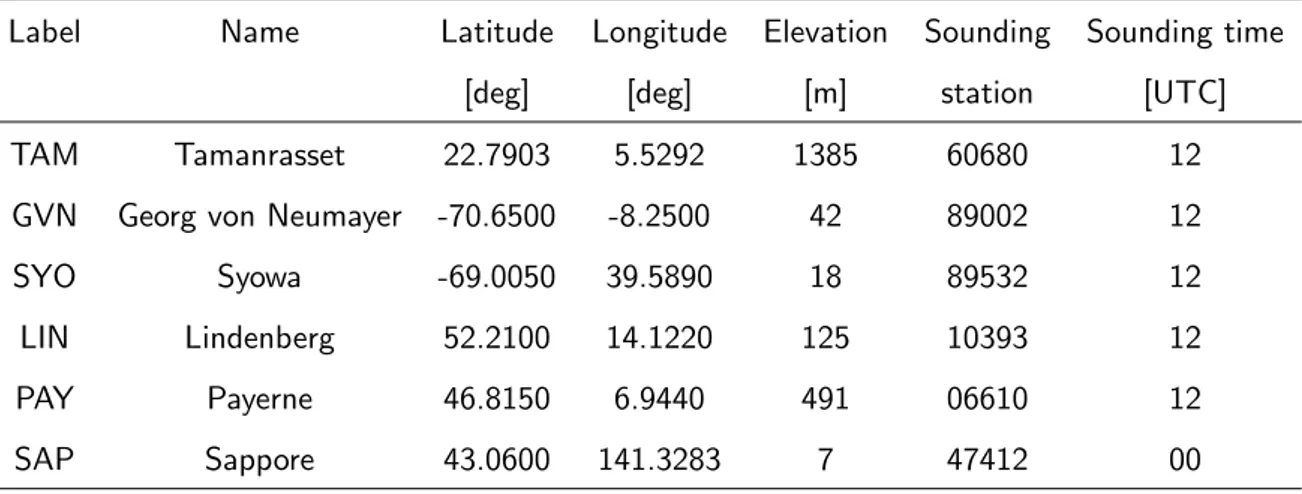
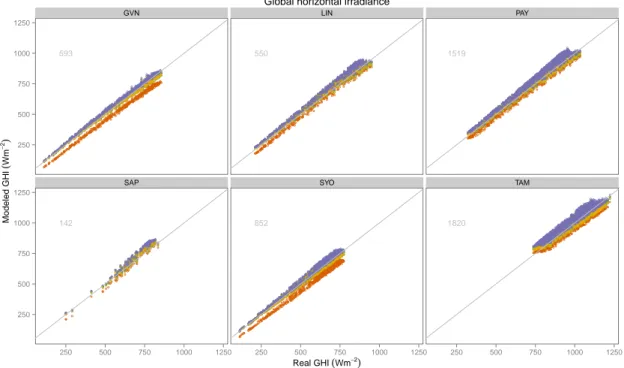
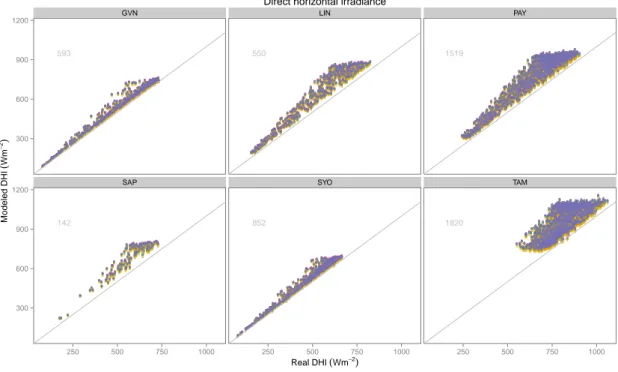
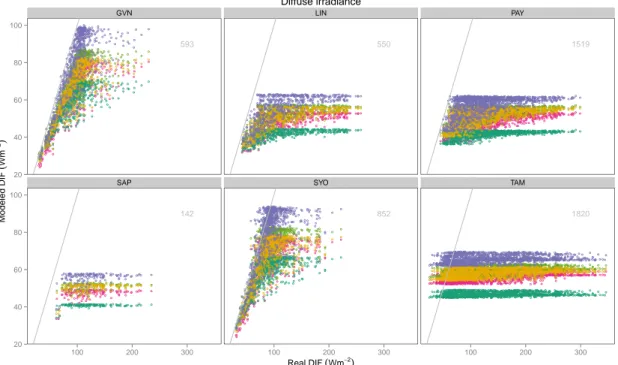
Documenti correlati
The results from the regular event history mod- els without fixed effects show that, net of the effects of all control variables, separation rates are lower while CFC is received,
But given that Norway has both a more egali- tarian wealth distribution and a more generous welfare state than for instance Austria or the United States, the question arises whether
Maria Antonietta Zoroddu, a Massimiliano Peana, a Serenella Medici, a Max Costa b. Department of Chemistry, University of Sassari, Via Vienna 2, 07100,
We identify the causal effect of lump-sum severance payments on non-employment duration in Norway by exploiting a discontinuity in eligibility at age 50.. We find that a payment
Finally, Model 4 includes all independent variables at the individual level (age, gender, having a university education, being a member of a minority group, residing in an urban
Per quanto attiene alla prima questione si deve osservare che Olbia conosce, in linea con precocissime attestazioni di importazioni romane già in fase punica l20 ,
A castewise analysis of the violent practices against women would reveal that the incidence of dowry murders, controls on mobility and sexuality by the family, widow
Come l’Herakles tebano ottenne dal dio Apollo delfico la promessa del- l’immortalità a patto che, terminate le fatiche impostegli da Euristeo, si trasfor- masse in oikistès inviando